Any bridge spanning a navigable waterway is inherently at risk for being struck by errant waterway vessels. Due to the large volume of vessel traffic on many waterways, such impact events are relatively common, with a catastrophic bridge collapse occurring, on average, once every three to five years in the U.S. An example of such an event occurred in 2002, when an aberrant two-barge flotilla collided with an interstate highway bridge near Webbers Falls, Oklahoma. The collision resulted in the collapse of a 503 ft section of the bridge, fourteen deaths, several million dollars in damage, and the need to reroute high-volume traffic for more than two months.
To address incidents of this type, the American Association of State Highway Transportation Officials (AASHTO) has developed design specifications which address the threat to bridge structures posed by large errant vessels. The AASHTO specifications treat vessel impact design in a probabilistic manner, wherein the probability of structural collapse is computed and checked against permissible limits.
Our research group, in a partnership with the Florida Department of Transportation (FDOT), has focused on the development of new analysis and design procedures intended to directly address complex topics in barge-bridge impact design that are not handled rigorously by current design provisions. In order to do this, a careful coupling of analytical and experimental investigations have been conducted, headlined by full-scale barge impact experiments conducted near St. George Island, Florida.
In 2004, full-scale barge-bridge impact experiments were conducted on a decommissioned causeway bridge near St. George Island, Florida. In the experiments, a full-size tanker barge was used to impact one of two different stand-alone piers, as well as a pier with the superstructure (bridge deck) in-place. Each pier was impacted multiple times, producing moderate levels of deformation in the barge bow. From the collected force and deformation data, it was discovered that, during the impact events, the dynamic response of the bridge pier amplified the peak impact force levels–a behavior not captured by the equivalent static procedures implemented in most bridge design codes.
Following the St. George Island tests, our research group developed a series of design-friendly analysis procedures which consider the barge-bridge interactions that cause dynamic load amplification during impact events. These procedures range from rigorous time-history analysis to simplified equivalent static procedures which implicitly consider dynamic effects. A revised probability expression to predict structural collapse due to barge impacts was also developed through an extensive study using these methods.
In addition to advanced analysis procedures, our group has used high-fidelity finite element modeling and simulation techniques to develop an impact load-prediction model that may be used in barge-bridge impact design. Numerous full-scale barge crushing simulations, conducted to develop the load-prediction model, showed that both bridge pier geometry and pier width can have a profound influence on barge impact forces–a consideration not currently incorporated into design standards. Force and deformation data quantified using analytical studies compare well with moderate-level deformation data obtained from the full-scale St. George Island barge impact experiments. However, to produce data for analytical model validation at high deformation levels, an additional experimental investigation was conducted.
In 2014, high energy barge impact experiments were conducted by our research group at the FDOT pendulum impact facility in Tallahassee, Florida. In each test a reduced-scale (40%) replicate of a jumbo hopper barge bow was struck by a steel-encased concrete impactor, representative of a bridge pier. To evaluate the influence of pier shape on barge force-deformation behavior, two separate test series were conducted–one with a round nose (circular) impactor, and one with a flat-faced (rectangular) impactor. Complementing the experimental effort, high-resolution impactor and barge bow finite element models were developed at the same reduced-scale (40%) as the test program and used in an accompanying analytical study. Data quantified from the numerical impact simulations of the specific impact experiments agreed well with collected experimental data, validating–at high deformation levels–the barge impact load prediction model.
Research Reports
2016
Determination of Barge Impact Probabilities for Bridge Design [ PDF file ]
The barge base aberrancy rate used in the AASHTO bridge design specifications was recalibrated using barge-bridge collision data and barge traffic data specific to Florida waterways. Potentially reflective of changes that have taken place in the barge towing industry since 1990 (e.g. advances in navigational technology), the recalibrated barge aberrancy rate was considerably smaller than the value currently specified by AASHTO.
2014
Validation and Implementation of Bridge Design Specifications for Barge Impact Loading [ PDF file ]
Reduced-scale barge impact tests were conducted to validate modeling and simulation techniques used in previous analytical investigations at high deformation levels. Experimental data were compared to results from high-fidelity finite element analysis, showing that both bridge pier geometry and pier width can influence peak force levels.
2010
Vessel Crushing and Structural Collapse Relationships for Bridge Design [ PDF file ]
A previously developed UF/FDOT barge impact load prediction model was updated to account for oblique impact scenarios. A new relationship was also developed to predict the probability that a bridge will collapse due to vessel collision. Cutting-edge probabilistic simulation techniques were paired with previously developed dynamic impact analysis methods to develop the new probability of collapse expression.
A Static Analysis Method for Barge-Impact Design of Bridges with Consideration of Dynamic Amplification [ PDF file ]
Dynamic amplification phenomena in bridge piers impacted by barges were investigated and characterized. A design-oriented equivalent static analysis method was developed that empirically accounts for dynamic amplification effects during barge collision.
2008
Development of Improved Design Provisions for Barge Impact Loading [ PDF file ]
Leveraging lessons learned from full-scale impact experiments, several new barge impact design and analysis procedures were developed. These included a new impact force prediction model, three dynamic impact analysis methods, and a numerically efficient bridge modeling technique. Where possible, the new methods were validated against previous experimental measurements.
2006
Barge Impact Testing of the St. George Island Causeway Bridge – Phase III:
Physical Testing and Data Interpretation [ PDF file ]
Fifteen full-scale barge-bridge impact experiments were conducted on a decommissioned bridge. Results were compared to finite element simulations of the experimental impact conditions, with good agreement.
2003
Barge Impact Testing of the St. George Island Causeway Bridge – Phase II:
Design of Instrumentation Systems [ PDF file ]
Instrumentation systems were developed to be used during full-scale barge impact experiments on a decommissioned bridge. Expected measurements from the proposed sensor systems were computed based on finite element simulations of the experimental.
2002
Barge Impact Testing of the St. George Island Causeway Bridge – Phase I: Feasibility Study [ PDF file ]
The feasibility of conducting full-scale barge impact experiments on a decommissioned bridge in Apalachicola Bay, Florida was investigated. High-resolution finite element simulations were used to predict impact forces and determine optimal parameters for the experimental test program.
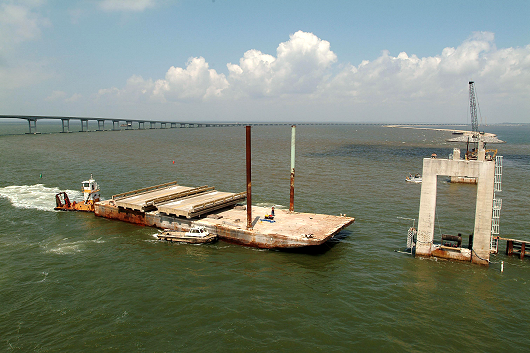
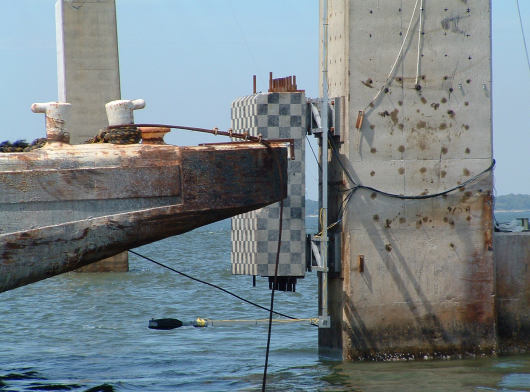
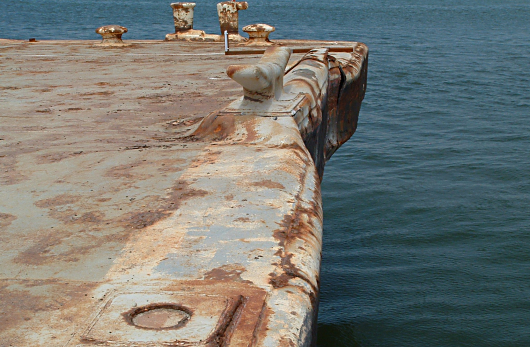
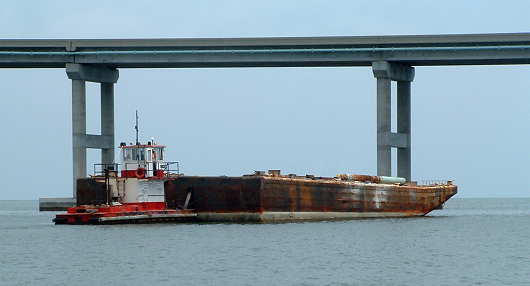
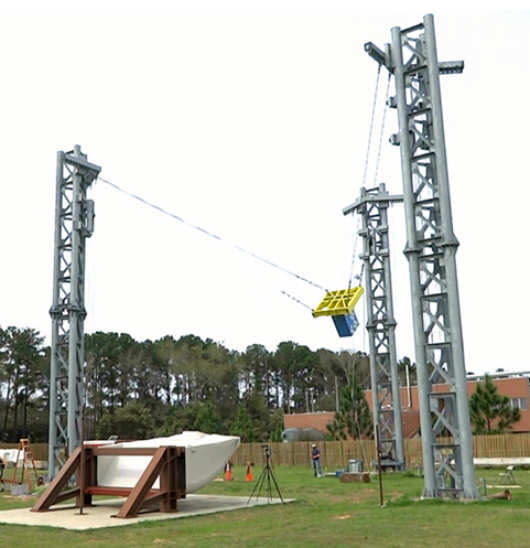
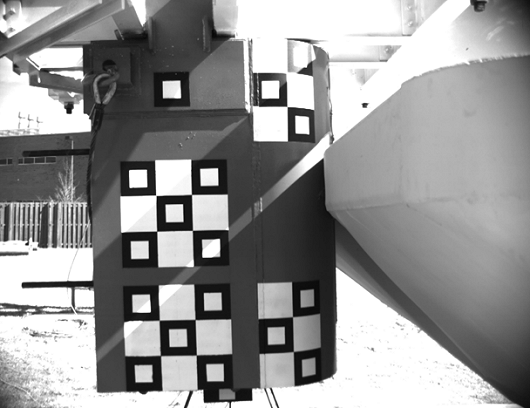
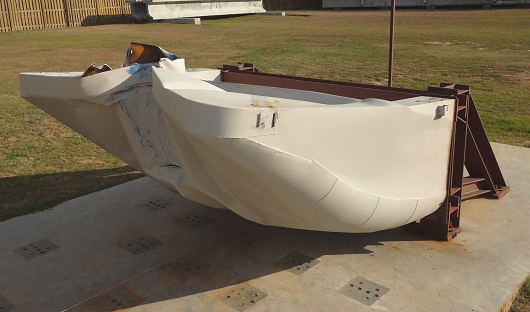
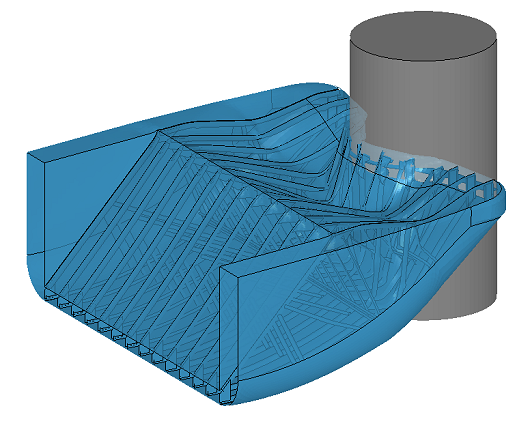
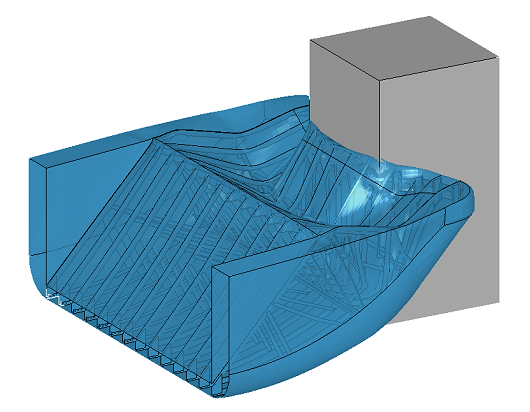
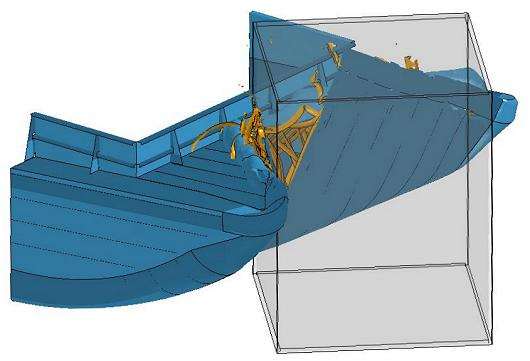
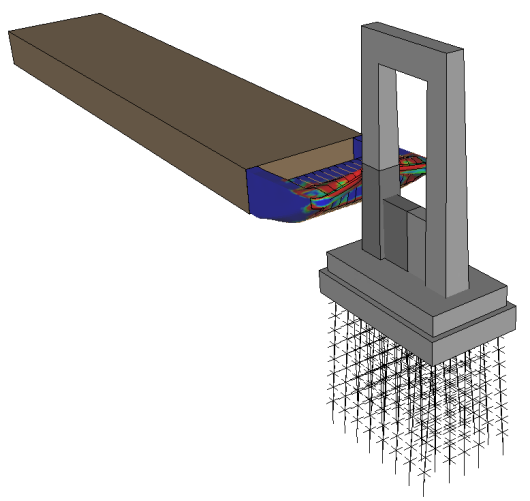
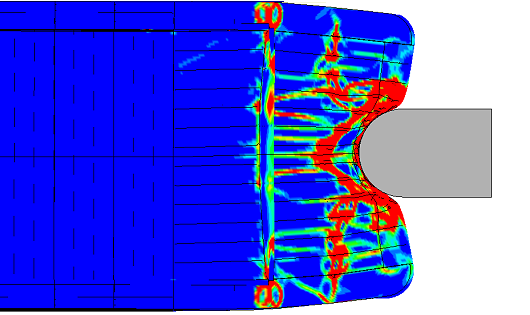